Smart quantum technologies
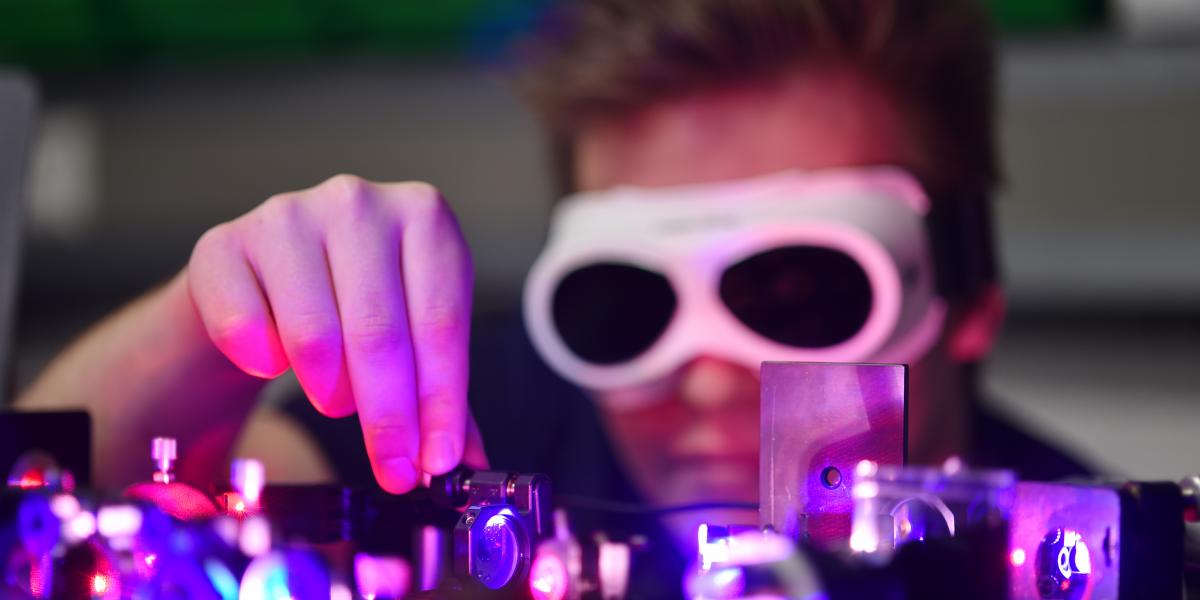
Our innovative developments are the basis for smart quantum technologies: We develop lasers and components for quantum generation, address production techniques and develop complete modules and systems for quantum technologies. We are working on new fiber-based components, innovative concepts for optical waveguides, and automated assembly. In this way, we want to establish compact and industrially suitable applications for quantum technologies in the future, such as quantum computers, quantum sensors, or quantum communication.
Your contact person
Dr. rer. nat. Andreas Wienke
Overview of smart quantum technologies
Lasers for quantum technologies
Lasers are enablers for quantum technologies. Novel, smartly designed light sources form the basic framework for a variety of quantum optical processes. We use our extensive knowledge in laser development, for example, for the generation of entangled photons and for cooling atom traps, thus expanding the technological basis for quantum technologies.
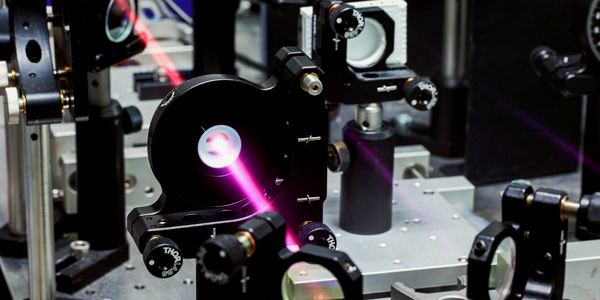
Single photons or entangled photon pairs are necessary for a variety of quantum optical applications. Novel measurement methods, such as quantum frequency comb interferometry, enable previously unattainable sensitivities of sensor systems. The key to this are ultra-narrowband pulsed laser systems. We use mode-locked laser systems with spectral bandwidths of a few gigahertz. These are coupled to nanophotonic micro-ring resonators to efficiently generate entangled photon pairs in a four-wave mixing process. We also deal with other excitation wavelengths in the visible near-infrared and infrared range (VIS-NIR-IR).
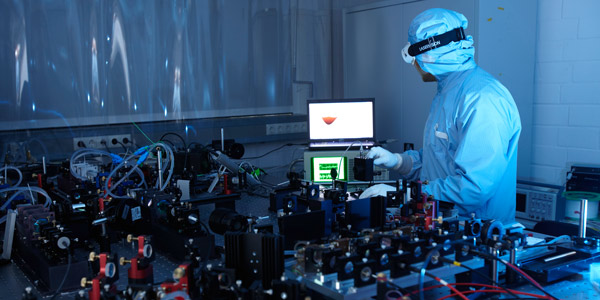
For dipole traps, we are further developing robust and powerful, continuously emitting fiber lasers in the range of around 2 µm, which have already been successfully tested under microgravity in drop tower experiments. For laser cooling and atom interferometry, we are working on both single-frequency, efficient, diode-pumped solid-state laser sources and frequency-doubled narrowband fiber laser systems with output powers of several 10 W at 780 nm, for example, which can be used for cooling of rubidium atoms, for example.
Production technologies for quantum technologies
We do not only develop and investigate components and systems for quantum technologies, we also work on bringing our know-how on optical production techniques more strongly into quantum technology applications. With our approaches and methods, we want to make quantum systems more compact and cost-effective.
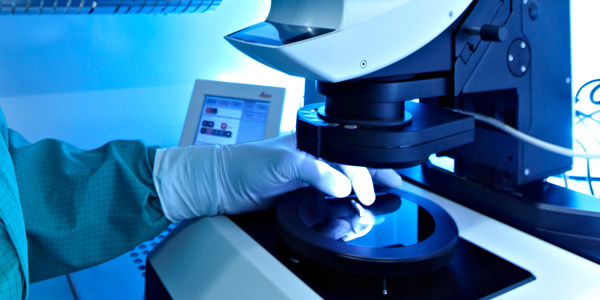
For highly integrated photonic systems, the precise assembly of the individual components is necessary to develop low-loss devices. We are working on methods to automate this for an industrial-suitable use. Furthermore, we deal with chip handling and fiber coupling in this context. We are also working on methods for optical and electrical contacting of optoelectronic components and high-resolution active and passive alignment. For direct quality assurance, we perform optical beam characterization and optimization during assembly.
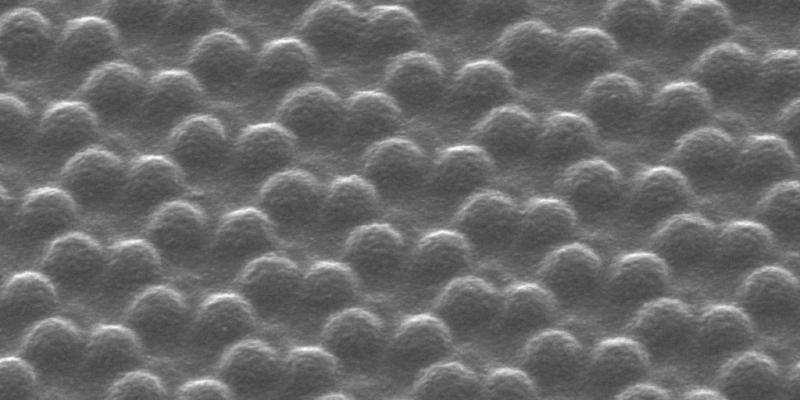
Micro- and nanophotonic patterning is the key to efficient quantum optical devices and components. Two-photon polymerization can be used to produce generatively fabricated polymer waveguides to create resonant, phase-matched structures. Such printed mode-field converters between different components (on-chip interconnects) reduce coupling losses and thus increase efficiency. We are also working on laser structuring processes for piezo-based tunable quantum emitters or microstructured ceramics for atom traps.
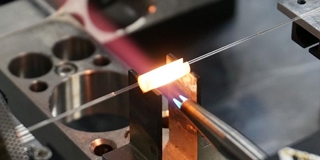
With high-precision fiber processing, we ensure that as few single photons as possible are lost within the optical components. Therefore, we are working on optimized splicing processes to connect the transitions between different components and fiber paths almost loss-free. We use CO2 lasers for processing cladding and core structures, which allows us, to generate long-period gratings (LPG) for mode conversion of single photons to achieve higher transverse quantum states.
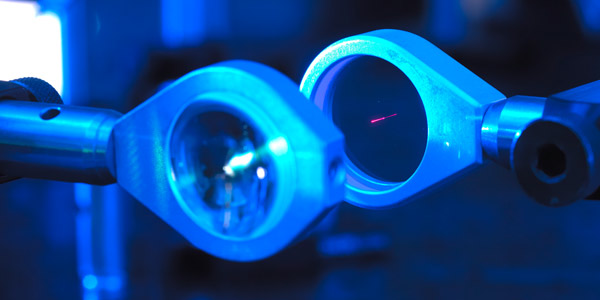
For decades, we have been pioneers in the development of optical coating systems for the highest requirements. We develop customer-specific coatings and perform characterizations on in-house and third-party developments. We work on layer transfer processes that enable the fabrication of self-supporting coatings and miniaturized filters. We are also involved in the fabrication of nonlinear photonic materials that can serve as active optical switches, for example. If special physical material properties such as defined band gaps and refractive indices are required, we tailor the necessary quantum-optimized layer systems.
Quantum-photonic components
We investigate optical components designed explicitly for quantum systems. For this purpose, we work on optimized fiber and waveguide components and combine them with our knowledge of nonlinear materials to fabricate micro-integrated quantum components. Our research is intended to lay the foundation for the industrial application of quantum technologies.
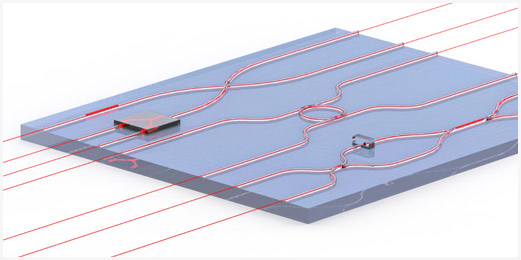
We manufacture fiber and waveguide components that are specially adapted to the requirements of quantum technologies and are constantly improving them. For this purpose, we are working on monolithic fiber components without additional splices and with very low losses. We are also involved in the simulation and fabrication of mode field converters for different fiber and waveguide geometries in order to create low-loss connections between different components. Fiber-based transverse mode converters can also be used to generate complex quantum states. Furthermore, we manufacture waveguides with customizable phases using 3D printing.
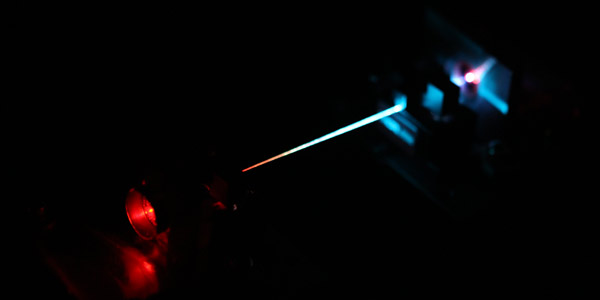
To increase the integration level of quantum systems while maintaining high efficiencies, existing quantum photonic concepts with a large number of discrete individual components must become significantly smaller and more compact. Only then, these systems can achieve industrial suitability. Micro-integrated quantum components as a hybrid solution offer many advantages for this purpose, such as low losses and easy interconnectivity using classical optical fibers. Furthermore, our miniaturized, self-supporting layer systems directly integrate additional optical functions such as signal filtering, optical switches, or even phase control.
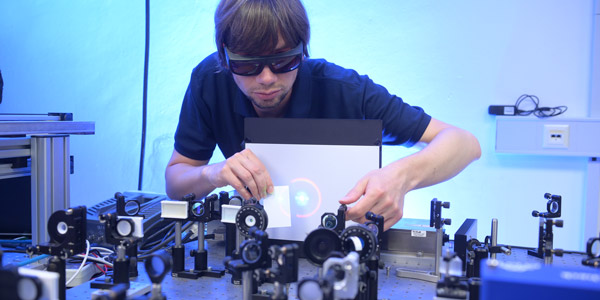
Nonlinear photonic materials impart new properties to optical components. We dealt with tailored bandgaps and refractive indices by quantum nanolaminates. We also design layered systems for the efficient generation of entangled photons. We also use nonlinear material properties to make light switching in optical layers only a few micrometers thick possible. We are working on continuously lowering the switching thresholds.
Modules and systems for quantum technologies
From individual modules for generating entangled photon pairs by spontaneous parametric down-conversion to complete fiber-networked systems and quantum light sources, we investigate key technologies for quantum engineering and prepare them for industrial use.
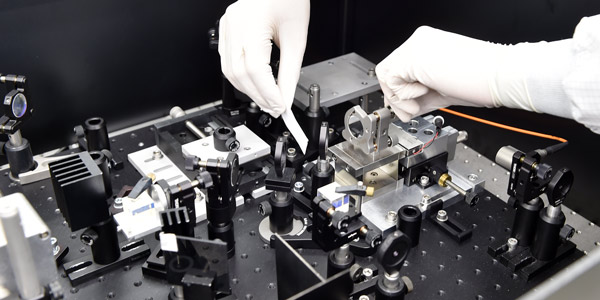
A variety of quantum optical applications can be realized with entangled photon pairs. These entangled photon pairs can be generated in different ways, such as polarization or frequency entanglement. The development of fiber-coupled modules with plug-and-play operation is currently the focus of our research, as these pave the way for quantum technologies to enter industrial use.
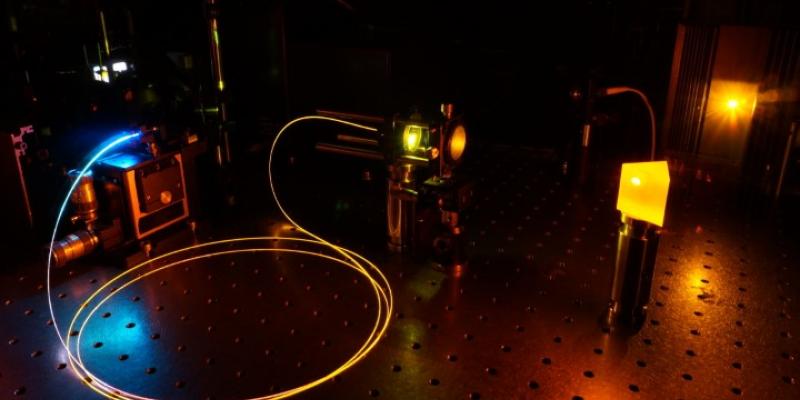
When signals are forwarded in fiber-optic networks, information is lost. This is particularly critical for the transmission of single photons over long distances. We are therefore working on low-splice fiber networks to minimize these losses and at the same time connect the components, modules and light sources - for this we use our many years of expertise in fiber technology.
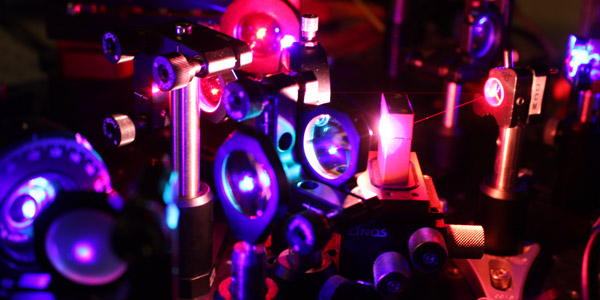
To make quantum computing, quantum internet, and quantum sensing industrially viable, highly integrated quantum light sources are needed that generate a large number of entangled photons in special states. We combine different fabrication processes such as ion beam sputtering, molecular beam epitaxy, two-photon polymerization, fiber coupler fabrication and automated assembly to approach this goal and fabricate scalable quantum light sources in the future.